US-Japan fusion materials collaboration marks 40 years of progress
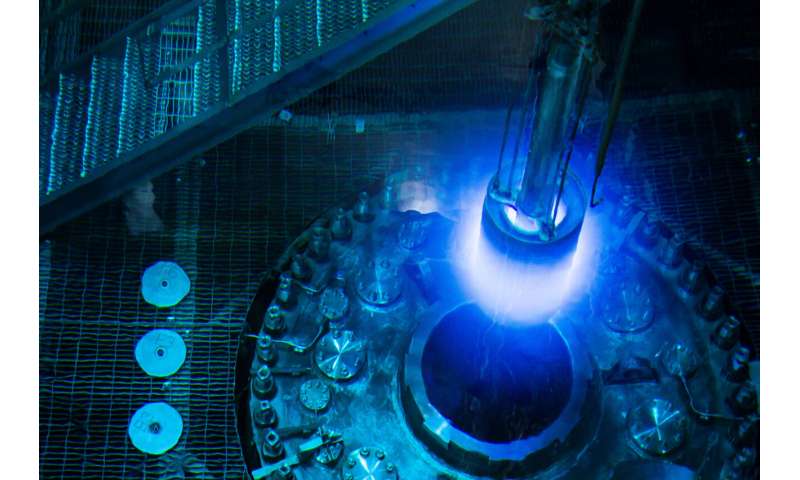
Creating energy the way the sun and stars do—through nuclear fusion—is one of the grand challenges facing science and technology. What's easy for the sun and its billions of relatives turns out to be particularly difficult to replicate on Earth.
On Earth, scientists must generate, confine and sustain a superhot gas called plasma—heated to 10 times the temperature of the center of the sun—to cause a fusion reaction. Although terrestrial plasmas can be confined magnetically, what materials can withstand near such high temperatures and the relentless impact of energetic neutrons? That question is central to the development of economical fusion power plants to provide abundant and carbon-free energy.
Scientists at the Department of Energy's Oak Ridge National Laboratory have been working with Japanese scientists under the Japan-U.S. Fusion Cooperation Program for decades to determine the answer.
"Materials development poses one of the greatest technical challenges for deploying a commercial fusion energy economy," said Yutai Kato, director of ORNL's Materials Science and Technology Division. "Of course plasma physics is important, but you have to have the materials that can withstand the harsh environment of fusion reactors. It's an extremely challenging requirement."
This requirement is so challenging that even after four decades of collaborative research among two generations of scientists in many countries, "at the moment, we don't have confidence in any materials that can survive in the fusion environment of a high flux of neutrons and extreme heat long enough to make fusion energy economically competitive," Kato said.
New materials—and the associated supply chains—will be needed for commercial and utility-scale fusion power plants to be viable.
For more than 40 years, the U.S. and Japan have been collaborating on joint scientific projects that attempt to determine the best materials for future magnetic confinement fusion energy machines, power plants based on tokamak or stellarator concepts. These collaborations use some of the world's best research tools, including a fission test reactor and radiological materials characterization facilities at ORNL.
"The Joint Projects under the Japan-U.S. Fusion Cooperation Program started in 1981 and continue to investigate a wide range of fusion materials and engineering issues, especially neutron radiation effects on these materials," Kato said. ORNL's High Flux Isotope Reactor, or HFIR, and other U.S. test systems have been the primary instruments to help elucidate effects of neutrons on materials. Kato added that he knows of no other scientific collaboration that has continued for that long. He and co-authors described the program in a paper in the Journal of Nuclear Materials last year.
The collaboration is defined by two distinct programs. One is a fundamental science program that has involved researchers at multiple Japanese universities and several U.S. laboratories. It started in 1981 and expanded to include ORNL in the 1990s to make use of HFIR. The second program features collaboration between ORNL and Japanese scientists at a government lab in Japan now known as the National Institutes for Quantum Science and Technology, or QST. That program began in 1983 with irradiation experiments, first in the Oak Ridge Research Reactor, then in HFIR.
The experiments were aimed at developing materials to use in experimental and demonstration devices and "the eventual power systems that we hope will evolve from these programs," said Bill Wiffen, an ORNL consultant. Wiffen was a member of the ORNL research staff for 25 years until 1989 and then was program manager for DOE fusion materials in the Office of Science for 10 years. He has been part of the collaboration for many years.
There are, however, candidate materials for fusion power plants, and scientists are eager to continue the collaboration. According to Kato, the research has identified three classes of materials that are promising for future fusion system applications: reduced activation ferritic/martensitic steels, oxide dispersion strengthened steels and silicon carbide composites. Another material, tungsten, is a favored candidate for specialized applications to withstand high heat and particle flux levels.
"We're working on the structural materials that have an international consensus of having the best chances of working," Wiffen said. "The steels look promising. We also have some hopes that tungsten will work as a plasma-facing material, and that's a focus of the fundamental program right now."
But none of the experimental fusion machines—including the international ITER facility in France, which will deliver long pulse operation for minutes at a time—can produce the high exposures to neutrons the materials scientists and engineers need. That's why HFIR is so important and "why we need a dedicated fusion neutron source. That's the number one new facility priority in the fusion community right now," Kato said.
The Material Plasma Exposure eXperiment, or MPEX, a DOE Office of Science project managed by ORNL slated for completion late this decade, will complement HFIR by providing the ability to test materials for use in the extreme plasma environments found in fusion energy devices.
Although the QST collaboration focused on designing and developing the materials and generating the qualification and licensing data for an eventual power plant, "for us, the value of that collaboration is to really connect our science program with the engineering program on their part so we stay practical and realistic," Kato said.
The university collaboration makes use of facilities throughout the U.S. and Japan with programs such as RTNS-II, FFTF/MOTA, JUPITER, JUPITER-II, TITAN, PHENIX and now FRONTIER.
Japan has an experimental tokamak and stellarator, but neither was planned to produce the neutrons needed for materials research.
In addition to these efforts with Japanese partners, ORNL also has growing collaborations with the European Union and the United Kingdom Atomic Energy Authority. "We hope these productive collaborations will continue," Wiffen said. "The results are measures of the synergistic values of working internationally to mature this important developing 21st-century technology."
Among the most important impacts of early U.S.-Japanese studies was a determination that the neutrons of fission and fusion reactions, which occur at different energies, result in comparable damage in terms of primary defects in the material. The collaboration then focused on other topics, including the dynamic irradiation effects under variable conditions, material system issues for blanket concepts, and materials and technologies for plasma-facing components. In addition to the relatively fundamental studies, the Joint Projects contributed largely to the development of candidate steels as well as ceramic composites and materials like tungsten.
In April 2023, Japan announced a national fusion strategy to help create an industry based on developing and commercializing fusion power. It thus join an international race that was already steaming full speed ahead. Fusion's advantages are so enticing that it is attractive to governments and now private investors alike. As a measure of this interest, investors in the U.S. are pouring in $5 billion to commercialize the process as a solution to climate change. But their fusion devices' success will depend largely on having the right materials.
Mickey Wade, associate laboratory director for ORNL's Fusion and Fission Energy and Science Directorate, said energy from fusion will be necessary if the world is to meet global carbon reduction goals.
"The projected worldwide demand for carbon-free electricity is surging," Wade said. "The only way to meet that demand is through nuclear fission and fusion energy playing a large role in our future energy mix."
Kato, who first visited ORNL as a student more than 30 years ago because of the collaboration and then joined the ORNL research staff about 10 years later, added, "We just have to figure out the right materials to get started."
Provided by Oak Ridge National Laboratory