Covering 30 years of fusion-energy research at EPFL
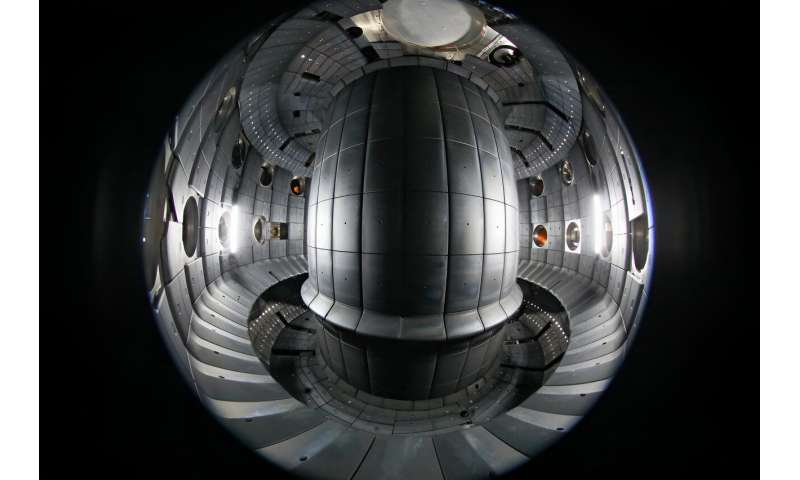
The intense heat and pressure inside stars, such as the sun, cause pairs of atoms, especially hydrogen atoms, to come together or 'fuse'. When these light nuclei merge into a heavier nucleus, some mass gets lost and is converted into a massive amount of energy, following Einstein's famous formula, E=mc2. This fusion process is what powers stars and releases an incredible amount of energy into the universe.
Scientists are already able to produce nuclear fusion reactions on Earth. The current challenge faced by researchers around the world is to sustain these fusion reactions continuously and harness the energy released in an efficient and controlled manner to generate electricity. At EPFL, the engineers have chosen to study a method that involves using a torus-shaped magnetic confinement reactor, called a tokamak.
In this approach, deuterium gas, a hydrogen isotope, is heated to 100 million degrees Celsius to transform it into a plasma and induce highly energetic collisions between the deuterium nuclei. The tokamak's magnetic fields suspend the plasma in the middle of the vacuum chamber and away from the interior wall of the device.
The Swiss Plasma Center, now with a staff of about 200 researchers and students, started up its own variable-configuration tokamak 30 years ago. Owing to its unique design, this experimental reactor has become one of the most important nuclear-fusion research facilities in Europe.
"We built the reactor even before there was the internet, and its core is still the same," says Basil Duval, a senior scientist working on the tokamak's measurement systems.
He points out that the research being carried out at the Swiss Plasma Center is known internationally—partly because of its contribution to the International Thermonuclear Experimental Reactor (ITER) project, and partly because the findings are of value to the entire nuclear-fusion research community. "For a country the size of Switzerland to have an experimental facility of this caliber is really outstanding," says Duval.
To commemorate the 30th anniversary of its tokamak, the Swiss Plasma will host representatives of the EUROfusion consortium in September. This consortium is behind a number of nuclear-fusion initiatives, including advancing the ITER physics basis and optimizing its chances of success via experimentation on facilities such as the TCV tokamak. Ambrogio Fasoli, director of the Swiss Plasma Center, is also the chair of EUROfusion and has just been named the consortium's program manager.
"Our work at the Swiss Plasma Center over the past 30 years has provided key insights into plasma behavior. The TCV plays a vital role in this endeavor. Recent upgrades to its infrastructure have expanded our capability to investigate key issues for ITER, DEMO, and future fusion reactors. The challenges ahead are substantial, but we are well-positioned to make significant contributions to the development of fusion energy as a critical component of the future global energy mix," he says.
A unique approach
Because EPFL's tokamak is a "variable configuration" reactor, scientists can use it to observe how changes in plasma configuration affect the plasma's properties (like temperature and confinement quality) and study new plasma configurations. It can also be used to evaluate different configurations for divertors, the devices used to control the release of energy from the reactor core. Their role is essential for being able to sustain plasma for long periods without damaging the reactor, and engineers are still working to optimize their design.
The Swiss Plasma Center recently teamed up with Google DeepMind to develop a new magnetic control method for plasmas, based on deep reinforcement learning, and successfully applied it to real-world plasma configurations in the TCV tokamak for the first time.
Like all tokamaks, the EPFL's tokamak features a vacuum chamber where gas is transformed into plasma. This chamber is encompassed by toroidal (torus-shaped) magnetic fields, created by large magnetic coils, that prevent the plasma from touching the interior wall of the chamber.
Additionally, there is a central column with ohmic coils that maintain plasma stability, and a poloidal field that shapes the plasma configuration. The entire reactor is outfitted with a heating system that utilizes microwaves and hot-particle injection, complemented by a comprehensive array of instruments that measure temperature, density, radiation, fluctuations in plasma configuration, and other vital parameters.
In future fusion power plants, the heat generated by fusion reactions within the plasma will power turbines-similar to current nuclear fission reactors-and produce substantial amounts of dependable baseload electricity. This process will be sustainable and carbon-free, without generating long-lasting radioactive waste.
Provided by Ecole Polytechnique Federale de Lausanne