Meet the JILA Postdoc and Graduate Student Leading the Charge in a Multi-Million-Dollar Quantum Sensing Project
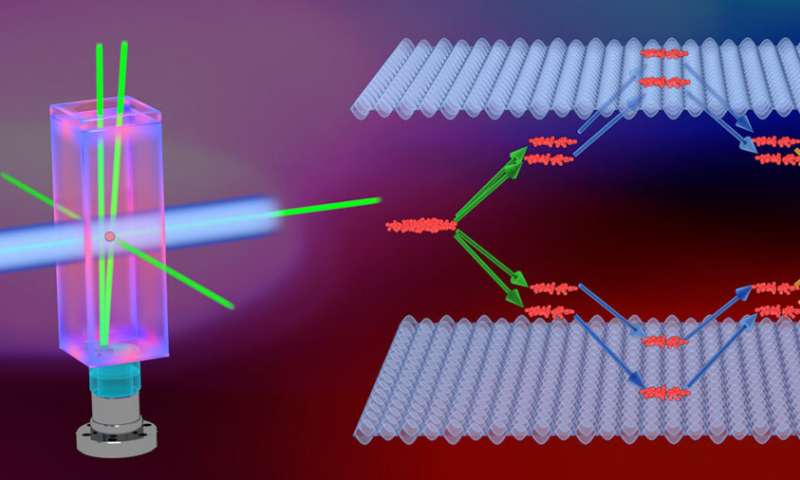
In the quiet halls of the Duane Physics building at the University of Colorado Boulder, two JILA researchers, postdoctoral research associate Catie LeDesma and graduate student Kendall Mehling, combine machine learning with atom interferometry to create the next generation of quantum sensors. Because these quantum sensors can be applied to various fields, from satellite navigation to measuring Earth's composition, any advancement has major implications for numerous industries.
As reported in a recent article preprint, the researchers successfully demonstrated how to build a quantum sensor using atoms moving through crystals made entirely of laser light. They applied accelerated forces to atoms along multiple directions and, using this sensor, measured the results, which closely matched values predicted by quantum theory. LeDesma and Mehling also showed that their device could accurately detect accelerations from just one run of their experiment, a feat that is very difficult to accomplish with traditional cold atom interferometry.
The experimental setup involved a sophisticated arrangement of lasers and other optical devices to manipulate and measure atoms at ultracold temperatures. Their approach was initially met with skepticism by many in the scientific community due to the novelty of the design and absolute reliance upon nonintuitive machine learning algorithms.
"Many thought that this type of measurement wouldn't work," explained JILA Fellow and University of Colorado Boulder Physics professor Murray Holland, the project's principal investigator at CU Boulder. "But the data is convincing."
While the scientific community may still be coming to terms with the potential of LeDesma's, Mehling's, and Holland's project to merge AMO (Atomic, Molecular, and Optical) physics with machine learning, the unique approach has already secured a $15 million grant from NASA as part of the Quantum Pathways Institute (QPI). The Quantum Pathways Institute is centered at the Center for Space Research (CSR) in Austin, Texas, and is led by Srinivas Bettadpur and colleagues.
Other collaborators in the Quantum Pathways Institute in Boulder include JILA Fellow and CU Boulder professor of physics Dana Anderson, professor of aerospace engineering Penina Axelrad, associate professor of electrical engineering Marco Nicotra, and group lead for the Sources and Detectors Group at NIST (National Institute of Standards and Technology) Michelle Stephens.
This institute is NASA's first step in exploring the potential advantages of quantum metrology over classical sensors deployed in space. As the QPI website states, the quantum sensors will be placed "aboard satellites in orbit around Earth to collect mass change data—a type of measurement that can tell scientists about how ice, oceans, and bodies of water are moving and changing." Such technology would lead to more accurate pictures of the effects of climate change and global warming on Earth.
Taking the Quantum Reins
Because their complicated nature can often span longer than the time of a Ph.D., many research projects within the physics community are inherited by a graduate student from a graduating doctoral student. In LeDesma's case, there was no prior graduate student to hand over a working experiment to her when she started on the project as a new graduate student in the fall of 2019.
"My introduction to the world of quantum gases was with a magneto-optical trap, or MOT," she said. "However, to do any of the precision metrology we wanted, I needed to learn how to make a Bose-Einstein-Condensate, and there were no students in the lab who could pass on that prior knowledge."
Considered the fifth state of matter, Bose-Einstein-Condensates (BECs) are composed of dense, ultracold clouds of gaseous atoms in the quantum regime. They have a long history at JILA, as JILA and NIST Fellow Eric Cornell and former JILA Fellow Carl Wieman were awarded the 2001 Nobel Prize in Physics for experimentally creating the first BEC.
When LeDesma arrived at JILA, just before the COVID-19 pandemic, she began to work on the BEC quantum metrology project that had formed the core doctoral work of a former graduate student Carrie Weidner, under the supervision of Professor Dana Anderson. Both Anderson and Weidner had spent years previously developing a special type of quantum metrology known as shaken lattice interferometry, which uses controlled vibrations of a grid-like structure to measure and study the behavior of particles precisely.
The BEC quantum metrology experiment was (and still is) housed in the Duane Physics building C-wing, an area susceptible to vibration, noise, and temperature variation, all unfavorable conditions for a sensitive precision experiment.
"For about a year and a half, I had to teach myself a lot of basic experimental skills. In that time, I completely rebuilt the 780-nm laser system necessary for cooling and trapping rubidium atoms and making BEC on an atom chip using RF evaporation. This experience proved instrumental for our future work which involved moving to a newer method of achieving BEC," LeDesma explains.
In 2021, Mehling arrived as a new graduate student in the laboratory. Together, LeDesma and Mehling made great strides in their BEC quantum sensor apparatus, including significant changes from the 2017 project. This included completely rethinking both how the experiment creates BECs and the method of performing interferometry itself, which involved a full experimental redesign and construction.
Together, they applied a contemporary method to evaporatively cool atoms with an all-optical sequence, successfully observing their first BEC with this method in the fall of 2022.
All-optical evaporation is performed within tightly focused, high-intensity laser beams. When two of these laser beams intersect, their crossing forms an optical trap where large numbers of hot atoms can be stored and collide with each other. Forced evaporative cooling is then performed by reducing the power of the laser beams. This process boils off the hot atoms similarly to the release of steam from a hot cup of coffee as it cools.
Using an all-optical method, the researchers do not need to use any magnetic fields during the cooling process, enhancing the BEC's potential size and stability. Additionally, this design allows the BEC to be more easily transferred into an optical lattice—a structure formed by interfering laser beams that create a standing potential of light. This lattice acts like a "light crystal" that can hold and manipulate atoms in a very controlled manner, enabling their use in interferometry experiments.
Engineering Your Own Quantum Sensor
Interferometers have a long, rich history as precision metrology instruments. Optical interferometers operate by splitting, mirroring, and recombining coherent light beams, resulting in discernable phase shifts in the light's interference pattern because of minute differences along the two paths. Measurement of these phase shifts can reveal information about the environment, and enable the sensing of inertial signals such as accelerations, rotations, and gravity gradients. These measurements form the basis of commercial gyroscopes such as ring laser gyroscopes and fiber optic gyroscopes.
More recently, AMO physicists have invested considerable research into atom interferometry, where the role of light and matter are effectively switched—that is, light pulses are used to split, mirror, and recombine the matter waves of neutral atoms to measure slight changes in their environment.
LeDesma, Mehling, and Holland further modified this approach, performing interferometry while the atoms interact with an optical lattice during the entirety of their sensing sequence. Dynamic control of the lattice phase (i.e., the location of light and dark regions) according to machine-learned protocols allowed the researchers to realize the necessary interferometry components.
At the sensor's core is a custom double MOT system built by Infleqtion, a quantum technology company. Within this ultrahigh-vacuum chamber, a combination of magnetic fields and precisely tuned laser light damp the motion of rubidium atoms with decelerations of up to 1000 m/s2, or 100 times the force of Earth's gravity.
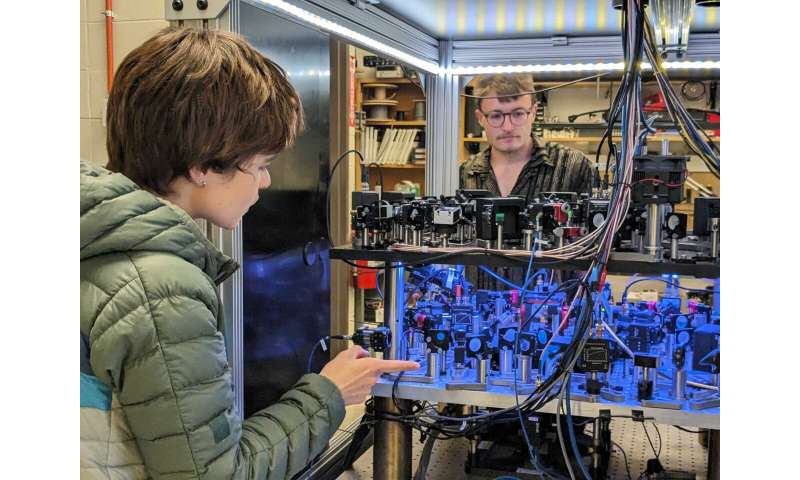
This cooling is achieved through Doppler cooling, a process in which lasers tuned to a color that is a slightly lower frequency than the color that the atoms typically absorb. This causes atoms to slow down when they move against the direction of the laser light. This laser force is strong as it reduces the temperature of the atoms by a factor of 10,000 in a few milliseconds.
In addition to the cooling effect of the laser light, magnetic field gradients are applied to exert a position-dependent force on the atoms. Combined, this process, known as a magneto-optical trap, or MOT, is a critical first step in many AMO experiments for quantum science.
In this common setup, the apparatus produces ultracold atoms in two distinct regions. In a lower glass chamber, dispensed atoms are initially cooled along two dimensions, and a laser push beam is used to propel the atoms vertically up through a tiny tube. They then appear and are recaptured in an upper chamber which has the pristine vacuum quality necessary to perform experiments where confining atoms for seconds is desirable.
"We produce a MOT in the bottom cell, and then we push atoms up to the 3D science chamber, where we load a three-dimensional MOT that would look maybe the size of a thumbnail," Mehling elaborated.
The 3D MOT typically accumulates one billion cold rubidium atoms over a five-to-ten-second load time. Additional cooling is achieved by changing the chamber's magnetic field strength and further detuning the laser light from atomic resonance.
The cold and dense atoms that result are then captured by intense 15-watt laser beams to perform all-optical evaporation to degeneracy. The brightness of the beams, and therefore the trap depth, is slowly lowered to cool the atoms below the critical temperature necessary to form a BEC. For a typical experimental run, LeDesma and Mehling produce 100,000 BEC atoms at an effective temperature of below 10 nK, 300,000 times colder than the initial MOT atoms.
Following these numerous cooling steps, the BEC is then loaded into their multidimensional optical lattice. While many experiments use retroreflecting mirrors to create their optical lattices because it is easier, LeDesma and Mehling instead use counterpropagating laser beams. This design offers greater versatility and flexibility in controlling the location of the lattice nodes and antinodes but is harder and requires meticulous alignment.
"It's like taking two strands of hair and overlapping them, and they have to align perfectly to create your lattice," Mehling added. "It becomes more complicated because our lasers aren't visible light; they're in the IR (infrared) spectrum, so we can't see them with the naked eye and have to use IR cards to detect where they are and then align them manually."
Adding AI to Quantum
The complexity of controlling pure quantum states like a BEC in an optical lattice involves numerous variables, each of which can drastically affect the outcome. The researchers employ sophisticated machine design techniques such as reinforcement learning (RL) and quantum optimal control (QOC) to develop and optimize the control sequences that manipulate the atomic wave functions within the lattice to perform interferometry.
Holland and his former graduate student Liang-Ying Chih developed much of the initial theory that the current experimental procedures for interferometry rely on. They applied reinforcement learning, a form of machine learning and artificial intelligence, to the quantum design problem and showed that atom interferometry could be done in this manner.
Additionally, LeDesma and Mehling worked alongside associate professor of electrical engineering Marco Nicotra and his former graduate student Jieqiu Shao to implement quantum optimal control algorithms to generate an alternative class of interferometry solutions. High-fidelity solutions obtained through both approaches demonstrated a successful interface between atom interferometry and computer programming.
The researchers experimentally apply these machine-generated solutions by changing the relative phase of individual lattice beams as needed.
The lattices' counterpropagating design enables the relative phase to be adjusted by inputs from a control computer. This means that controls can be changed on the fly, and the sensor's behavior can be changed at will by software programming rather than by modifying the experimental hardware. This programmability of their sensor allows the device to operate as either an accelerometer in one dimension or a multi-dimensional gyroscope via the flick of a virtual switch. However, initial experiments wielding this additional flexibility proved nontrivial.
"There was quite a learning curve to go through to understand how to apply the machine-learned protocols and to get the BEC atoms to do precisely what we wanted" explained Holland. "But we are now so good at it that if we find a great solution on the computer, we know with high confidence that we will be able to apply it to the experiment."
The final component of their sensing sequence involves the detection and imaging of the atoms. A resonant probe aligned with each lattice axis enables absorption imaging of the atoms following lattice phase modulation. Absorption images of the atoms provide detailed information about the momentum and position of the atoms, allowing the researchers to reconstruct the atomic wavefunctions and measure the atoms' response to inertial signals.
In practice, the matter-wave interference pattern from which a signal is extracted is seen by allowing atoms to fall under gravity and separate into discrete momentum components. The relative occupation of atoms in these multi-order momentum states changes with an applied inertial signal. Imaging the atoms after they have undergone interferometry and time of flight enables the researchers to reconstruct the applied signals.
In their most recent experiments, the sequencing of the machine-learned protocols allowed the experimental team to perform a special type of measurement known as two-dimensional Michelson interferometry, which is used in other apparatuses that do not involve machine learning.
LeDesma and Mehling revealed the resulting 49 momentum diffraction peaks in the atoms' 2D interference pattern, which had not previously been seen by other experiments. Measurements of the many-order diffraction pattern provided the critical information needed to determine the magnitude and direction of external accelerations. This information was crucial for calibrating the sensor's sensitivity, optimizing performance, and calculating the application's experimental stability.
To better understand the systems dynamics and predict their sensor's response and performance, LeDesma and Mehling worked closely with JILA Fellow Murray Holland.
"This project works because we've been working heavily with the theorists, who can help translate all the protocols," Mehling added. "Murray was here sitting in the lab with us, trying to get the control protocols to work from the beginning of the project. He comes down to the lab and gets his hands dirty and I don't think we could oversell Murray's importance and how good of an advisor he is."
While Holland's research has been in quantum theory, his 30-year history of studying BEC dynamics allowed him to help guide the implementation of these novel interferometry sequences and realize the importance of their research.
"It's impressive what Catie and Kendall have been able to achieve in such a short time," Holland commented. "They quickly recognized what problems had to be solved and found solutions. The result is work that is opening a whole new avenue of research which will develop into a substantial field, with JILA at the forefront."
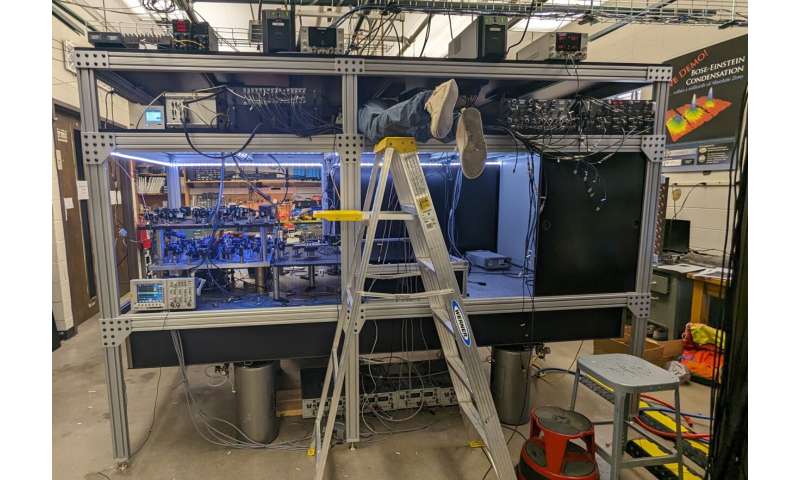
NASA Buys In
In March 2023, the BEC quantum metrology experiment got a significant boost in funding with the help of the NASA Quantum Pathways Institute grant, a $15 million grant over five years to several universities working on applying remote quantum sensing to study the Earth's climate.
As CU Boulder has been designated as the testbed site for this grant, LeDesma and Mehling are leading the charge, along with Holland, the lead PI for the Colorado portion of the grant, and with the other CU Boulder research collaborators.
Rebuilding a Laboratory in Seven Days
Away from JILA's dampening floors and piped-in purified water and air, the C-wing of the Duane Physics Building suffers from vibrations and thermal fluctuations from the glass windows and countless students walking, biking, and skateboarding past the lab on the concrete sidewalks and paths.
In the fall of 2023, LeDesma and Mehling, fed up with the older building's issues, decided to remodel the experiment, taking apart the entire apparatus and rebuilding it from the ground up.
"The temperature stability in this lab is horrendous," LeDesma elaborates, "The temperature swings up to five degrees daily, which can greatly affect laser beam pointing stability. In our original system, this caused drifts in the alignment of the light used for all-optical evaporation as well as our lattice beams used for interferometry. We were having to realign beams at least four to five times daily to combat this."
To compensate for these issues, LeDesma and Mehling worked with JILA's instrument shop to create a custom climate-controlled enclosure to supply cooled, filtered air to their experimental system. However, to install this new enclosure, the experiment had to be deconstructed. The full demolition day was August 15, 2023.
"Installation of the new enclosure required us to not only remove a majority of the optics off the table but also included removing all the electronics and other elements used to control the experiment. We had to uninstall all the rack units that originally held all of these components above the optics table." LeDesma says. "After we installed the new enclosure, we started putting optics back on the table. It took us six days to obtain our first BEC following the rebuild."
The rebuild took seven days, not because of external pressure but because of LeDesma and Mehling's efficiency in working with their system, which they had reconfigured dozens of times.
Their experimental rebuild has proven instrumental in the researchers' abilities to conduct interferometry experiments. With the enhanced stability, the team can go months at a time without anything more than slight alterations to the experiment, consistency LeDesma and Mehling hope to leverage as they continue to explore the potential of their sensor.
Skeptics Emerge
While combining AI's machine learning processes with the sensitivity of quantum mechanics could produce the next generation of quantum sensors, not all are convinced by the results of these experiments.
The standard point of view is that if a scientist wants to measure things precisely, they will want their atoms in the dark and not interacting with anything, a notion popularized by the 1989 Nobel Prize winner Norman Ramsey in his method of separated oscillatory fields.
But here in the lab, the atoms always see the light crystal that they move in, and so the environment must be perfectly controlled. As upgrades to the experimental system are installed and the team continues to report improved performance metrics, Mehling believes more experimenters will be willing to adopt some of their strategy and design philosophies.
In March of 2024, Holland presented the accelerometry results from the one-dimensional and two-dimensional experiments for the first time at a CU Boulder Physics Department Colloquium. For both LeDesma and Mehling, the feeling was one of validation.
"We finally had some significant results to show that this thing actually worked," Mehling says. "After the talk, people came up and asked questions. Later, more people visited the lab, wanting to understand what we were doing. We have been very isolated in Duane, and it's nice to feel that way no longer."
Moving Towards Greater Sensitivity
Now, with an improved setup, a NASA grant, and possible future collaborations with other JILA researchers, LeDesma and Mehling are interested in pushing the boundaries of their apparatus as much as possible.
"We want to turn this into a precision measurement experiment," Mehling adds. "Currently, it's not as sensitive as we need. We have an end-user for our experiment, goals, and a timetable to keep progress moving sustainably."
With its versatile design, the researchers have proposed that the apparatus can potentially measure various phenomena, from Earth's gravity and tidal distribution to the detection of dark matter.
"The realization of the fact that matter can behave as waves and interfere constructively and destructively lies right at the core of the development of quantum mechanics more than one hundred years ago," explains Holland. "And so, atom optics systems are very fundamental, and to show that we can control them exquisitely with modern machine learning and optimization methods introduces a disruptive solution to a very old technology. We think this experiment is now placed to have a bright future—full of potential—and we don't know at this point how far we will be able to take it."
More information:
Catie LeDesma et al, Vector Atom Accelerometry in an Optical Lattice, arXiv (2024). DOI: 10.48550/arxiv.2407.04874 , arxiv.org/html/2407.04874v1
Provided by JILA