The natural direction of heat flows—from hot to cold—can be reversed thanks to quantum effects
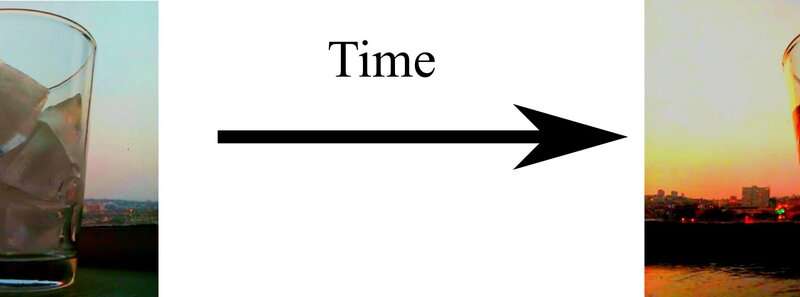
In a hot summer Sunday braai or shisa nyama (South African barbecue), if you don't finish your favorite beverage quickly, the ice cubes will melt and the drink will get warm—undoubtedly spoiling your braai/shisa nyama. That's because the natural direction of the heat is always from hot to cold (Fig. 1). The reverse, a drink that grows increasingly colder—which would definitively save your Sunday—looks like science fiction. Indeed, this would be like reversing the arrow of time. Our recent study establishes that things might be very different in the quantum world.
The ice cubes' fate in Fig. 1, summarized by the formula "the heat always flows from hot to cold," is a consequence of the second law of thermodynamics. Then, considering a quantum system interacting with its environment assumed to be initially hotter, the heat flow's direction is from the environment to the quantum system, as for your favorite drink. Things change when the quantum system is in a state presenting genuine quantum properties called quantum coherences. In particular, such quantum coherences are responsible for coherent superpositions, the central phenomenon around the famous Schrödinger's cat.
This deserves a short digression: Going back to the beginning of quantum mechanics, Schrödinger came up with a thought experiment aiming to highlight the unusual properties of the quantum world and to question its range of validity. Indeed, at the nanoscale, elementary particles can be found in coherent superposition of different states. For instance, an atom can be in a coherent superposition of excited state and ground state, which means that the atom is simultaneously in the excited state and in the ground state—fundamentally different from being either in the excited state or in the ground state.
According to the thought experiment designed by Schrödinger, this singular property of the atom could be transmitted (through a device activated according to the atom's state) to any object or, for instance, a cat. At the end of the experiment, the cat would be left in a coherent superposition of being dead and alive, a state analogous to the ground and excited state superposition of the atom. This cat became famous, and since Schrödinger himself did not give it a name, it is simply called the Schrödinger's cat. Obviously, we never saw, and will most probably never see, any cat in such confusing state. This apparent contradiction between the predictions of quantum mechanics and our everyday experience highlights the still very active debate around the transition between quantum and classical world and the underlying "measurement problem." However, small objects like electrons, atoms and molecules can really be found in such coherent superpositions.
Note that this has to be distinguished from the phenomenon of correlation, another fundamental aspect of quantum mechanics and physics in general. Two (or more) systems or objects are correlated when they share information about each other, in the sense that the knowledge of the current state of one object provides some information about the state of the other object(s). This happens, for instance, after interactions between the objects. This is because during the interaction, each object is being affected depending on the other objects' state.
As an illustration, we can use the example of a pair of atoms. Let us consider that one atom is initially in the ground state while the second is in the excited state. After letting them interact for some time, their state might have changed so that we no longer know their respective state. However, assuming that the pair remained isolated during the interaction, the excitation initially present in the second atom is still in the pair. Then, by measuring the first atom and finding that it is in the excited state, we can deduce that the second atom is certainly in the ground state. Conversely, if the first atom is measured in the ground state, we can deduce that the first one is in the excited state. Then the knowledge of the state of one atom gives us information about the state of the other—they are correlated. Indeed, they are classically correlated.
There exist quantum correlations that are stronger than classical correlations. Roughly speaking, perfect quantum correlations would allow us to answer any question about the second atom given that we already answered that same question for the first atom (by measuring it). By contrast, classical correlations allow us to answer only a predefined question, for instance, the energy (location of the excitation) in the above example. Differently from correlations, coherent superposition is always a genuine quantum phenomenon. The property we suggest using to reverse the natural heat flow between a quantum system and its environment is precisely coherent superposition.
Let's reconsider the simple example of a pair of atoms, and assume the atoms are initially cold and immersed in a hot environment (Fig. 2). The natural heat flow direction can be affected when the pair of atoms contains delocalized excitations, meaning excitations that are simultaneously in both atoms, in the same way the Schrödinger cat is simultaneously dead and alive. In such a situation, the pair appears as hotter, in some sense as if it was really not one excitation, but two—one in each atom.
This led us to define a concept of apparent temperature to describe how the environment "sees" the object. Consequently, even if each atom is individually colder than the bath, together, they can appear hotter than the bath, thanks to the delocalized excitation they share. Then, in such a situation, the pair starts releasing energy, getting colder and colder while heating the hot bath: The natural heat flow is reversed (Fig. 3). We derive precise conditions on both temperatures of the atoms and the environment, as well as the corresponding amount of quantum coherences (quantifying the "degree" of delocalization of the excitation) needed to reverse the heat flow.
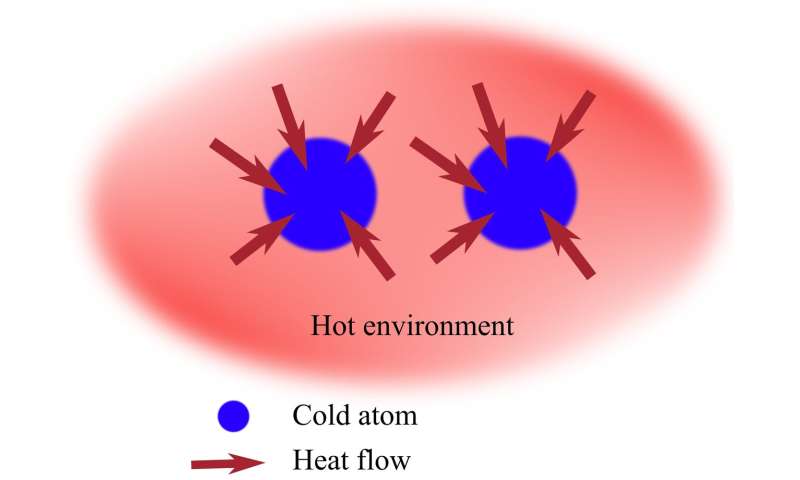
Remarkably, the complementary phenomenon is also possible: the reversal of the heat flow between a hot pair of atoms immersed in a cold environment. The required quantum coherences are slightly different—they have to be negative. This can be interpreted as destructive interferences related to a shared excitation. Namely, instead of being simultaneously present in both atoms as above, it is as if the excitation were "simultaneously absent of both atoms" (while being in the pair of atoms). Such states are sometimes called "dark states," because the excitation is never released.
We also show that heat flow reversal is not limited to pairs of atoms, but instead can take place in ensembles of atoms or spins, and more generally in any quantum system containing energy degeneracy—the existence of energy levels of same energy (remembering that quantum systems have discretized, or quantized, energy levels).
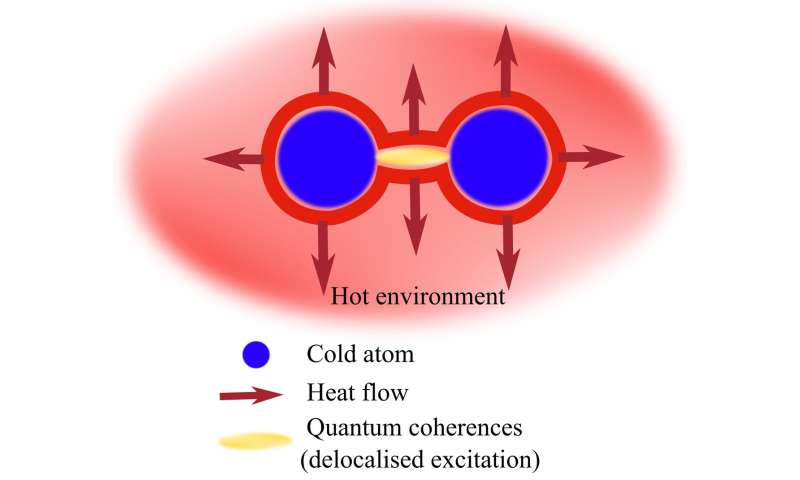
Before concluding, it is appropriate to mention that another phenomenon can give rise to heat flow reversal. This was even shown experimentally (at the nanoscale) between a cold and a hot atom. There, the underlying mechanism used to deceive the heat flow relies on having two atoms initially correlated. Then, this alternative heat flow reversal is not exclusively a quantum phenomenon: It works with both classical and quantum correlations, even though the later can lead to larger heat flow reversals.
Additionally, in the situation of an object immersed in a hot environment—your drink in the hot summer air—the necessity of initial correlations means that the object has to interact previously (and in a specific way) with the environment, which is not always realizable, practical or desirable—this could, for instance, damage your drink. This drawback can be avoided using quantum coherences, as we showed above. Moreover, this suggests that the heat flow reversal we put forward, being based on quantum coherences, has no classical equivalent.
We will have to wait a bit longer for ice cubes that get increasingly cold in the hot summer. Even if large-scale heat flow reversals are not realized anytime soon, this quantum heat flow reversal increases our understanding of energy and information exchange at the quantum level, which is fundamental for quantum technologies. This also illustrates the impact of quantum coherence on apparent temperatures. Moreover, the quantum effect pointed out in this study might trigger the discovery of additional quantum effects. It can also lead to innovations in energy production or refrigeration, at least at the nanoscale, which is becoming increasingly important, for instance, due to the ever shrinking size of electronic components in information and computation technologies.
This story is part of Science X Dialog, where researchers can report findings from their published research articles. Visit this page for information about ScienceX Dialog and how to participate.
More information: C. L. Latune, et al, Heat flow reversals without reversing the arrow of time: The role of internal quantum coherences and correlations, Phys. Rev. Research 1, 033097 (2019). DOI: 10.1103/PhysRevResearch.1.033097
E. Schrödinger, Die gegenwärtige Situation in der Quantenmechanik, Naturwissenschaften 23: pp.807-812; 823-828; 844-849 (1935). DOI: 10.1007/BF01491891
C. L. Latune, et al, Apparent temperature: demystifying the relation between quantum coherence, correlations, and heat flows, Quantum Sci. Technol. 4, 025005 (2019). DOI: 10.1088/2058-9565/aaf5f7
K. Micadei, et al, Reversing the direction of heat flow using quantum correlations, Nat. Commun. 10:2456 (2019). DOI: 10.1038/s41467-019-10333-7
Bio:
I am currently a postdoctoral researcher at the quantum research group of the University of KwaZulu-Natal, Durban, South Africa. I am a specialized in quantum information and quantum metrology (theory), and my current focus is on quantum thermodynamics: the study of thermodynamics at the quantum level. Myself and my collaborators are quite active in this exploding field, with 6 recent publications in highly ranked physics journals. I did my undergrade and Master studies in Math and Physics in France (École Normale Supèrieure, Paris, France and Université Paris XI, France). I followed with a PhD at the Universidade Federal do Rio de Janeiro (Federal University of Rio de Janeiro), Brasil.