A new way to tackle the neutron lifetime enigma: A superfluid helium-4 scintillation detector
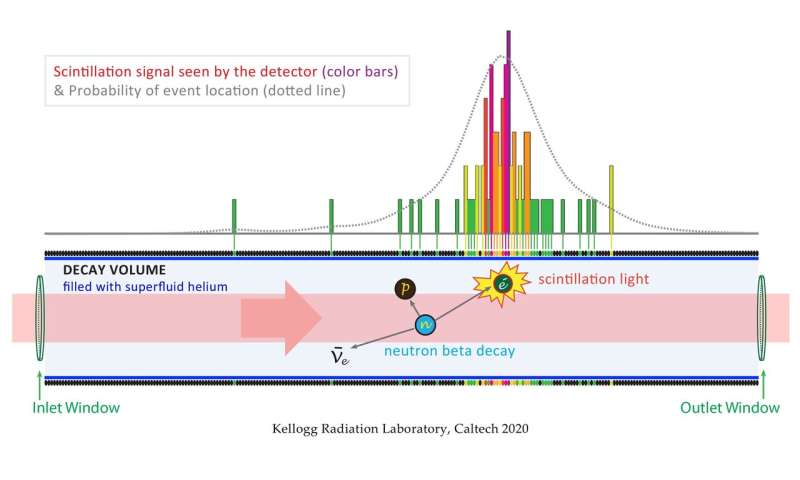
A free neutron outside a nucleus is not stable. It undergoes beta decay at a probability. Over time, the number of free neutrons decreases exponentially at a time constant, which is called the neutron lifetime.
What is the value of the neutron lifetime?
Do you have a feeling that you should've known it? So did I. It should have been mentioned in a middle-school textbook, perhaps in a corner one simply missed. Since the neutron lifetime is a fact of nature so fundamental and important to understanding the universe, people might assume it had been long settled. Yet it hasn't. To be fair, scientists have tried hard for decades via many high-precision measurements, but came back with two completely different values in two classes of experimental methods: 879.4 +/- 0.6 seconds by the bottle method vs. 888 +/- 2.0 seconds by the beam method.
The difference of eight seconds is as large as four times the measurement uncertainty of two seconds. It means the chance they would agree with each other is about 60 in 1 million, almost impossible. In the field, we call it the neutron lifetime enigma. Could it come from a miscalibration in experiments, or more excitingly, an implication of new physics?
The tales of two methods
In the bottle method, neutrons can be sealed in a vacuum bottle made of neutron friendly material or confined by magnetic fields and gravity. These neutrons have extremely low kinetic energy and move at speeds of a few meters per second. They are called ultracold neutrons (UCNs). Once in a while, you can open the bottle and count how many neutrons are still in there. After normalizing to the initial filled number, a series of neutron survival ratios with respect to different storage times can lead to a measurement of neutron lifetime.
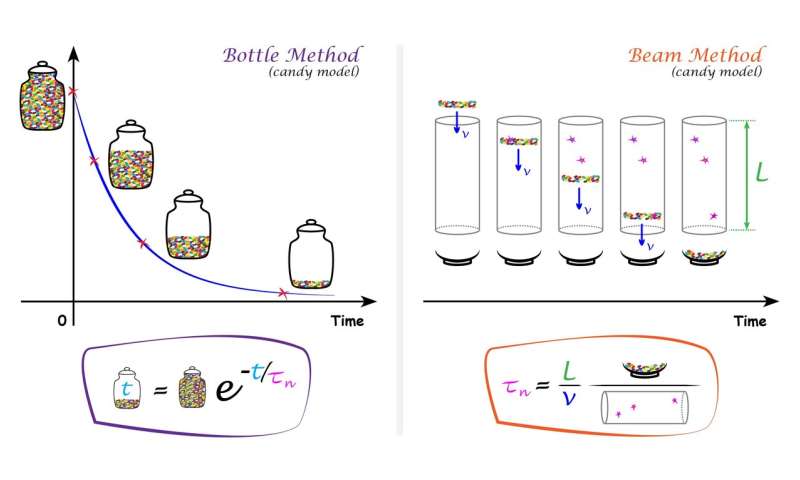
Across the aisle, in the beam method, neutrons are sent through a defined volume in the form of a particle beam. These neutrons move at speeds of several hundreds of meters per second, much faster than UCNs. They are called the cold neutrons (CN). You can count how many neutrons have undergone beta decay during the flight time they spent in the defined volume. After normalizing to the beam flux, the neutron death ratio during the time they spent in the given volume can also lead to a measurement of neutron lifetime.
So far, there are seven high-precision bottle results with various setups, but only two beam results. Both of these beam measurements used the same technique, called the Penning trap. The decay product, protons, are collected in the Penning trap and get counted by a well-calibrated detector.
There is no doubt that more beam experiments of more varieties are desperately needed to compare and check.
How to do it differently?
In the beam method, we detect how many neutrons have undergone beta decays. It has three decay products—a proton, an electron and an electron anti-neutrino.
Well, it's a bit difficult to detect anti-neutrinos, because their interaction cross-section to matter is so small. The world-leading neutrino detectors are often gigantic and targeting on an intensive flux source, such as the sun or a nuclear plant, but only see a handful of events each year. So anti-neutrinos do not represent a realistic option.
We then consider protons. So far, results with the best precision in the beam method were all obtained via detection of protons; an upgraded experiment called BL3 is under preparation at NIST, U.S. Thereafter, detecting electrons becomes appealing for a new beam experiment. Researchers at J-PARC recently announced their preliminary result of neutron lifetime via detecting the beta-decay electrons with a time projection chamber (TPC). It is a revival of an experiment first proposed by Kossakowski et al in 1989. They are currently working to improve precision.
After decades of efforts by generations of brilliant researchers, you might assume all plausible ways should have been thoroughly explored on the beam method.
Or have they?
I have a new proposal: How about using a superfluid helium-4 scintillator to detect the neutron decay product—an electron?
A crazy idea at first glance, but it will work
This looks unbelievable, as most of the neutron lifetime experiments are done in a high vacuum condition to eliminate neutron scattering on gas particles. An exception is the J-PARC experiment, where a working gas is required by TPC to amplify the charge of a beta-decay electron into a detectable current. Sophisticated analysis has to be applied to identify and remove the background events induced by the scattered neutrons.
If running a neutron beam through a gas is troublesome, why consider a liquid?
But it would work thanks to the amazing properties of superfluid helium, a quantum liquid. The liquid forms a macroscopic quantum wavefunction, and most of it condenses into the ground state. Elementary excitations in the quantum liquid are called phonons and rotons, which were famously predicted by Landau in 1947 and confirmed by inelastic neutron scattering.
Yes, neutrons do scatter within superfluid helium, but only on the elementary excitations. And a condition must be satisfied. That is the conservation of energy and momentum. Cohen and Feynman showed in their paper published in 1957 that the scattering never happens if the neutron wavelength is longer than 16.5 Angstroms. It means neutrons of low energy and long wavelength can penetrate superfluid helium-4 as if it were a high vacuum. The finding legitimates this proposal of a new beam experiment with a superfluid helium-4 scintillator.
Superfluid helium-4 as a scintillator
Superfluid helium-4 has been well studied as a candidate scintillation detector for neutrinos and dark matter. When charged particles of high kinetic energy impinge into superfluid helium-4, helium atoms will be ionized, excited and emit scintillation light. The process is quite complicated, but in general, the number of emitted photons is linearly proportional to the energy of the charged particle. The recoiling electron carries a kinetic energy in a range from zero up to 782 keV out of the released nuclear energy in the beta decay. The number of decayed neutrons thus can be counted via the frequency of scintillation.
In the meantime, the neutron flux of the pulsed beam must be monitored. This can be done with the isotope helium-3, which captures a neutron, converts into a proton and a triton, and releases 764 keV of energy. The rate of such capture events is proportional to the beam flux. These events are nuclei recoils. By contrast, the decay events are electron recoils. Therefore, the capture and decay events have a different set of signatures in scintillation signal. In the prompt glow, a capture event produces many fewer photons per unit of deposited energy than a decay event. The capture event has a short stopping range of tens of microns, whereas the decay event has a long track up to 2 centimeters. By analogy, one looks like a supernova, and the other looks like a meteor. In addition, they have a distinct behavior in the after-glow decay rate. With the scintillation detector, both the rates of decay and capture events can be simultaneously acquired in the same volume.
A road to high precision
To tackle the neutron lifetime enigma, high precision is the key. A new experiment is only meaningful if the precision can achieve 0.1% or sub-1 second.
It is almost impossible to register all the beta-decay electrons because a small portion of them have an energy too low to produce adequate scintillation light. But there is a solution. On the one hand, the proposed detector will provide a position resolution along the beam axis. Only the events in the central region will be used for high precision data analysis. On the other hand, we will collect as much light as possible. The detector is designed to cover more than 96% in solid angle for events in the central region, so that the energy of beta-decay electrons can be accurately reconstructed. A large number of these events compose a precise beta decay spectrum, which is well described by Fermi's theory of beta decay. The low end of the spectrum might be missing due to faint scintillation, but their number can be complemented by the fit of the precisely measured beta decay spectrum at higher energies.
Furthermore, suppression of background events is also important, especially those correlated with the scattered neutrons. The lack of neutron beam scattering on superfluid helium is already a good start. All the stray neutrons scattered from the windows of the volume will be captured by neutron absorbers surrounding the detector to minimize neutron activation.
In addition, the detector will also see Compton events induced by prompt gamma ray emission in the neutron captures on the inlet and outlet windows. It will appear as two bright bursts in time sequence and can be used as a timing and intensity reference for the position reconstruction of signal events, calibration of the detector and characterization of the beam spectrum.
New experiment, new opportunities
This new method is dramatically different from existing beam experiments. It doesn't need a strong magnetic field. It employs a pulsed beam with neutrons of a much lower energy. And a superfluid helium scintillation detector offers a distinct set of systematic effects. Of course, there are many technical difficulties to overcome. But I hope this new idea will eventually help resolve the neutron lifetime enigma and ultimately lead to new opportunities to discover new physics.
This story is part of Science X Dialog, where researchers can report findings from their published research articles. Visit this page for information about ScienceX Dialog and how to participate.
More information:
Wanchun Wei. A new neutron lifetime experiment with cold neutron beam decay in superfluid helium-4, Journal of Physics G: Nuclear and Particle Physics (2020). DOI: 10.1088/1361-6471/abacdb
Bio:
Dr. Wanchun Wei, an experimental expert in the frontier study of particles in superfluid helium. He obtained his PHD degree in physics at Brown University, and completed the postdoctoral study at Los Alamos National Laboratory. He is currently a Research Engineer at the Kellogg Radiation Laboratory, California Institute of Technology, USA.