Fluid dynamics of COVID-19 airborne infection suggests urgent data for a scientific design of social distancing
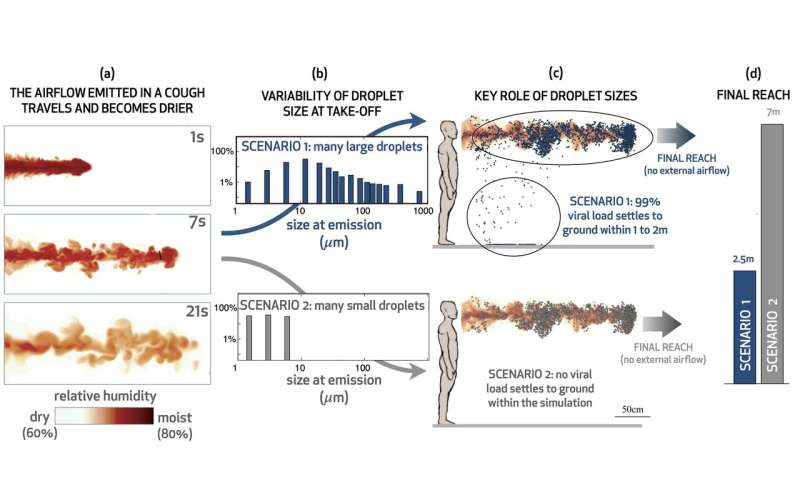
Infection by COVID-19 is largely caused by airborne transmission, a phenomenon that has rapidly attracted a great deal of attention from the scientific community. The SARS-CoV-2 virus hosted in different tracts of the respiratory system is emitted as we breathe, speak or sing or through more violent expulsions like coughing or sneezing. In these common actions, people emit thousands or even millions of small droplets of saliva acting as a vector for the virus. Given that the disease travels on respiratory droplets, social distancing is of paramount importance to limit the spread. Indeed, droplets are heavier than air, and sooner or later, they fall to the ground, which will tame their infectious potential.
Can we be more precise than "sooner or later?" As we unpack below, the reach of a droplet depends on its size. We all know from direct experience that when we speak, cough or sneeze, we often discharge large droplets: We can clearly see them and even feel them on our skin. But besides the visible droplets, we also scatter a myriad of invisible tiny droplets. This substantial variation in droplet size, from micron to millimeter, causes a great deal of uncertainty in determining the actual reach of the viral load expelled by an infected individual.
Are we certain to be safe if we keep one meter apart, as suggested by current guidelines?
If the subject is uncovered, the answer to this question is no: one meter is not a sound safety distance. To be clear, it is important to keep as far apart as possible, but we should not feel safe when standing one meter apart.
To understand why one meter is not enough, let us start from the beginning. The life of a respiratory droplet is dictated by the exact same physical processes that produce clouds. As cloud droplets are carried by the wind, they often encounter moist air and grow by condensation to become rain; we know all about the equations that describe both transport and condensation in clouds because they have been studied for centuries. Respiratory droplets undergo the same two physical processes, except respiratory droplets are carried by the air emitted in the cough and encounter dry air outside the mouth; thus, instead of growing, they shrink from their original size to their final size by evaporation.
Does droplet size matter? The answer is yes, and the reason is quite intuitive: Large droplets fall quickly, whereas small droplets fall slowly. As a consequence, smaller droplets linger in air for longer and may travel several meters before they finally reach ground. On the other hand, larger droplets travel less far in air, as they promptly reach ground. To follow the erratic path and shrinkage of the many diverse droplets from emission to landing, we used the equations from cloud physics for the two key processes of droplet transport and evaporation. Importantly, we could predict the fate of a droplet given its initial size when it first exited the mouth.
And here come the problems. We do not really know the typical size of the emitted droplets in a cough; some studies claim that the vast majority (97%) of saliva droplets are smaller than one micron in radius; other authors report evidence that only 45% of droplets are sub-micron in size. Others yet find no evidence of sub-micron droplets. Discrepancies may be partly explained by the use of different techniques, but it is also possible that there is an intrinsic variability, with different people and conditions causing droplets to shift in size. The scientific community is only now starting to study the fascinating physical processes that produce respiratory droplets. We just do not much about it yet. Having no reason to discard any of these data, we set out to compare the consequent scenarios.
Our strategy and results
We took advantage of one of the best high performance computing (HPC) facilities in the world, the Oakbridge-CX cluster at the Information Technology Center at the University of Tokyo, to which we had access thanks to the HPCI Urgent Call for Fighting against COVID-19 (grant hp200157), we followed in detail the evolution of thousands of droplets emitted in a typical cough. We compared scenarios that ensued from four experimental studies on droplet radius distributions. The four scenarios are remarkably different (see Figure 1): (1) the amount of viral content reaching the ground within 1–2 m varies from none to 99% of the total viral load. (2) Even in the absence of external wind or aeration, small droplets could travel from less than 2.5 m in one scenario up to more than 7.5 m in a different scenario.
Conclusion 1
The first conclusion of our work is that a major effort is needed to gain an understanding or at least a robust characterization of droplet size distribution in human expulsions. In the absence of more conclusive data, and despite the importance of social distancing, we are unable to predict what the safe distance is.
One more issue must be addressed
Intriguingly, our results show that disease transmission may also depend on the relative humidity (RH) of the environment. In dry conditions (RH lower than about 45%), droplets dry out and shrink to their crystallized salt core, similar to what happens as sea water dries out leaving solid salt on our skin. This process leaves the virions trapped onto the solid salt nucleus within a fraction of a second. In contrast, in moist conditions (RH larger than 45%), droplets never evaporate entirely and remain liquid at all times. The evaporation process is highly nontrivial, as humidity fluctuates widely due to turbulence as shown in the video for a typical cough (color coded according to the value of RH).
Are dry nuclei or liquid droplets more infective? This second issue is still debated, and no consensus has been reached. Imagine that SARS-Cov-2 absolutely needs water to survive. In dry days, disease transmission would be hindered and we would be much safer than in moist conditions. The question for social distancing would then be how far liquid droplets travel before complete evaporation, and we could interrogate the model described above to find the answer. We could also imagine the opposite scenario, where virions better thrive on solid nuclei and suffer in droplets, for example, due to the large concentration of salt or saliva. In this case, we would want to pay particular attention during dry days, and potentially keep indoors environments more moist.
Conclusion 2
- Humidity in the environment dictates the final state of the exhaled saliva droplets which either remain in a liquid state or reduce to their dry residual depending on the ambient relative humidity.
- A major effort is needed to define the infectious potential of the SARS-CoV-2 virus when transported on dry nuclei versus liquid droplets.
Populations worldwide continue to face severe restrictions to fight the current pandemic. Science is of paramount importance to unravel the physical and biological processes that dictate the path of respiratory droplets from take-off to landing and their potential to propagate infection. The formidable scientific effort we have witnessed in the past few months must continue: Only by joining forces will we be able to design effective guidelines for social distancing and provide a sound measure of safety that the public can rely on to make decision about personal behavior.
This story is part of Science X Dialog, where researchers can report findings from their published research articles. Visit this page for information about ScienceX Dialog and how to participate.
More information: M. E. Rosti et al. Fluid dynamics of COVID-19 airborne infection suggests urgent data for a scientific design of social distancing, Scientific Reports (2020). DOI: 10.1038/s41598-020-80078-7
Duguid, J. P. The size and the duration of air-carriage of respiratory droplets and droplet-nuclei. Epidemiol. Infect. 44, 471–479 (1946).
Yang, S., Lee, G. W., Chen, C.-M., Wu, C.-C. & Yu, K.-P. The size and concentration of droplets generated by coughing in human subjects. J. Aerosol Med. 20, 484–494 (2007).
Andrea Mazzino, Full professor of Fluid Dynamics at the University of Genova (Italy), PhD in Physics on turbulent transport, Physics Dept., University of Genova (1997).