Reactive molecular dynamics reveals fundamental mechanism of resistive switching in 2D materials
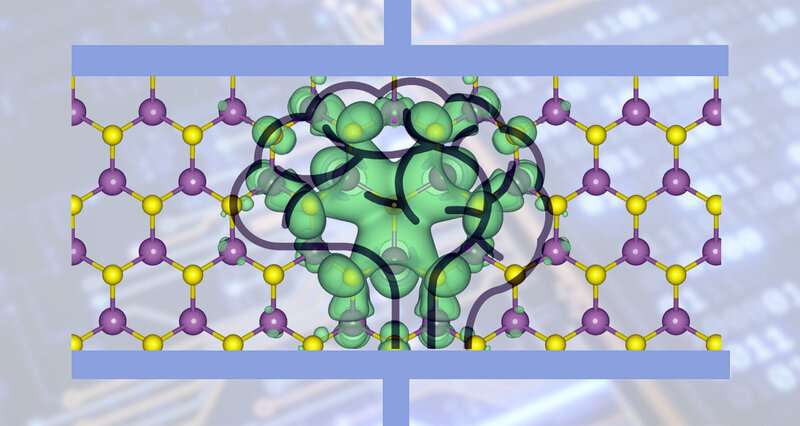
Conventional computers are based on von Neumann architecture in which data processing and storage are physically separated. Data shuttling between processing unit and memory leads extra energy consumption and restricts the processing speed. Recent progress in artificial intelligence technologies and neuromorphic circuits led scientists to overcome the data-shuttling bottleneck of von-Neuman architecture by creating brain like computing architecture where all the computation happens uniquely within the memory. Such computing paradigm is known as "in-memory computing" that can eliminate energy intensive and time-consuming data movement.
To implement the in-memory logic operations concept, non-volatile random-access memory (NVRAM) has been introduced. The storage principle in NVRAM is based on the physics of the active materials and the device where they are integrated, which is completely different from conventional random-access memory (RAM). NVRAMs come in the form of resistance switching RAM (RRAM), phase change memory (PCM), magnetoresistive RAM (MRAM) and ferroelectric RAM (FeRAM). These devices usually have two distinct physical states that can be switched between each other reversibly and rapidly under external bias.
RRAM consists of simple metal-insulator-metal (MIM) structure, in which resistance of the insulating layer defines the physical state of the device. In traditional RRAM devices usually bulk oxide materials like SiO2, TiO2, HfO2 and amorphous Si are used as active dielectric materials. In such devices low resistance state (LRS) is obtained by forming conductive filament due to migration of oxygen vacancies and metal cations of the bulk oxide layer or migration of metal ions from active electrodes under external field. As the direction of the field is reversed the filament ruptures bringing back the device in high resistance state (HRS). However, these bulk devices possess high switching voltage and long switching time and cannot be scaled down to atomic level.
With the emergence of two-dimensional (2D) materials, the recent past has seen a surge of experimental demonstrations of memristors, in which bulk dielectric layers are replaced with 2D materials. These 2D MIM structures can overcome the vertical scaling obstacle of the traditional RRAM devices and offer highly dense, fast, and ultra-low-power technology solutions. However, the underlying physics behind the ultra-fast resistive switching in such atomically thin devices is not well understood yet, even for an extensively studied material such as 2H-MoS2.
In our recent work, which is published in npj 2D Materials and Applications ("Theory of nonvolatile resistive switching in monolayer molybdenum disulfide with passive electrodes"), we investigate the origin of resistive switching (Rs) in a monolayer molybdenum sulfide (MoS2) based memristor-labeled as "atomristor."
We have used reactive molecular dynamics (MD) simulations and rigorous first principles-based calculations to atomically describe the RS phenomena in vacancy inhabited monolayer MoS2 with inert electrodes. Reactive force field used in MD simulation can model chemical reactions such as bond breaking and formation with an accuracy close to that of ab initio simulations with a much lower computational expense.
We discover that with the application of a suitable electric field at the vacancy positions, the sulfur atom from the other plane 'pops out' and gets arrested in the plane of the molybdenum atoms. To gain insight into the conductivity change because of S atom popping, we calculate the density of states (DOS) using density functional theory (DFT). Surprisingly, S atom popping causes the appearance of new states at and around the Fermi level, alluding metallic nature of the popped configuration.
From the energy-projected charge density for states at and around the Fermi level, we found the major contributions for the metallic states originate from the atoms situated within a 5 Å-radius circle (0.785 nm2 area) around the popped atom. This region can be termed as "virtual filament" since it functionally mimics the conducting filament of oxide-based memristors. From charge and bond-order analysis, we found that after popping the charge of the popped S atom double and the Mo–S bonds become significantly stronger than the normal Mo–S bond. Climbing image nudged elastic band (CINEB) calculations also show the reverse transition barrier from the vacancy to the popped state is significantly higher than the forward barrier. Because of much stronger bonds and an extremely high energy barrier, significant force is required to bring the popped S atom back to its initial location. Only reverse electric fields are not sufficient to bring back the atom. Combination of a suitable negative field and a high local temperature, which models the Joule heating due to high current flow in the conductive regions, restores the initial atomic arrangement.
According to our proposed theory the resistive switching mechanism in monolayer MoS2 based device is as follows:
The device is initially at high resistance state (HRS) as MoS2 in hexagonal phase is semiconducting in nature. As the electric field is increased to a certain value, the S atoms at vacancy centers pop into Mo plane creating metallic path and the device jumps into low resistance state (LRS)-referred as "SET." As the electric field is further reduced to zero the popped atoms remain at the same locations and the device continues to be in the LRS implying the memory operation. After applying a sufficient reverse field and localized heating the popped atoms move to their initial locations and the device retrieves its HRS-refereed as "RESET."
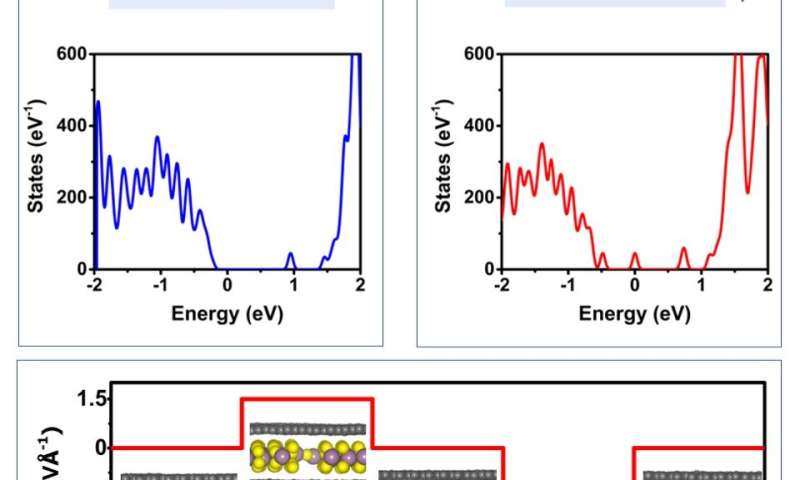
Some key successes of our proposed theory are:
- Our theory successfully explains almost all experimentally observed phenomena including bipolar and unipolar switching.
- The theory suggests that resistive switching is an intrinsic property of the S-monovacancy inhabited MoS2 and is independent of the electrode materials. Thus, it explains switching with inert electrodes like Graphene.
- The theory successfully reproduces the ultra-fast nature of the switching, achieving a complete SET-RESET cycle in tens of nanoseconds.
- The theory successfully captures the effect of localized Joule heating observed in experiments.
The insights provided can be useful for defect-engineering of MoS2 and even other 2D transition metal dichalcogenide materials for better memristive performances, which could lead to the realization of ultra-fast and reliable in-memory neuromorphic computing.
This story is part of Science X Dialog, where researchers can report findings from their published research articles. Visit this page for information about ScienceX Dialog and how to participate.
More information:
Sanchali Mitra et al. Theory of nonvolatile resistive switching in monolayer molybdenum disulfide with passive electrodes, npj 2D Materials and Applications (2021). DOI: 10.1038/s41699-021-00209-0
Bio:
Sanchali Mitra is an IoE (Institute of eminence) Post-Doctoral fellow of Indian Institute of Science (IISc) Bangalore. Arnab Kabiraj is a PhD student of Indian Institute of Science (IISc) Bangalore. Santanu Mahapatra is working as Professor at Indian Institute of Science (IISc) Bangalore. For more information see: santanu.dese.iisc.ac.in and nsdrl.dese.iisc.ac.in