Messy prebiotic chemistry may be key to homochiral life
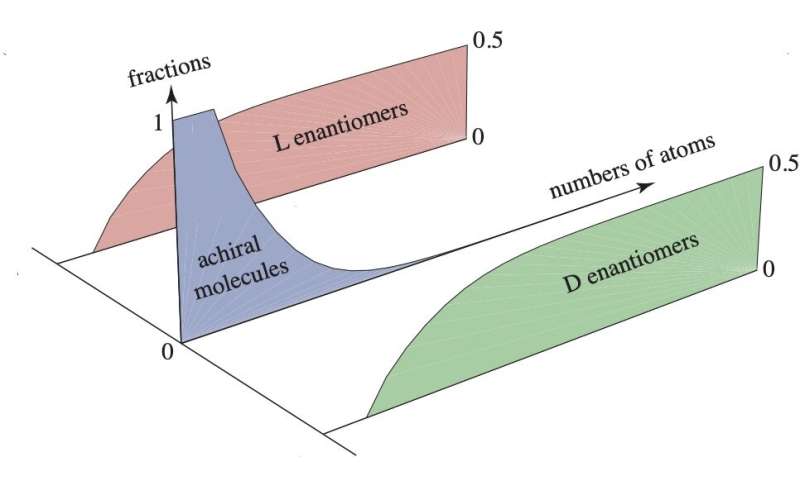
The word chirality comes from the Greek χǫιρ, which means hand. Chiral molecules form a pair of molecules that are each other's mirror images, similarly to our left and right hands. The chirality of molecules in the microscopic world was first discovered by Pasteur in 1853, who also observed that micro-organisms have a preference for molecules of a well-defined handedness. This observation was the first to suggest a possible link between chirality and life.
Today, we know that this form of chiral discrimination is general at the molecular scale. Among the two possible forms of a chiral molecule (enantiomers), only one of the two forms is present across the entire biosphere. For instance, all amino acids are right-handed; all sugars are left-handed in our cells. We call this phenomenon "homochirality." This homochirality has structural and functional implications at all length scales, from molecules to plants and organisms. Understanding the mechanism by which it has emerged is one of the long-standing puzzles in the research on the origins of life. Solving this puzzle may provide us with new insights or methods to detect possible life forms outside earth.
A new pathway to homochiral life
"As shown by F. C. Frank in the '50s, homochirality can be traced back to a symmetry breaking occurring in a non-linear chemical reaction network," explains P. Gaspard, professor at the Université Libre de Bruxelles. Once the symmetry is broken, the homochiral state can be maintained out of equilibrium thanks to a flux of matter or energy in the system. At thermodynamic equilibrium, such a flux may not exist and the only possible stable state of the system would be the racemic state, where both enantiomers are present in equal amount: Homochirality would be impossible.
"The novel idea of our work is that in a large chemical network, the chiral symmetry breaking is facilitated precisely because it is happening in a large network built by many species," says D. Lacoste, researcher at ESPCI, Paris. To support this idea, we first asked ourselves how abundant are chiral species within the chemical space of all possible molecules. This is something that G. Laurent, a Ph.D. student also working at ESPCI, Paris, quantified, thanks to a chemoinformatic analysis of a large chemical database, PubChem, which contains approximatively a total of 133 million molecules of known structure. He found that molecules longer than about 10 heavy atoms (any atoms except hydrogen) are significantly more likely to be chiral than achiral, confirming an earlier analysis of a numerically generated database, containing molecules which should exist according to the basic rules of chemistry.
"Now that we knew that there are a large number of chiral compounds, we focused on possible mechanisms that could lead to a preference of one enantiomer over the other one," adds D. Lacoste. In this limit, we could employ tools of random matrix theory to illustrate that the stability of the racemic state undergoes a phase transition as the number of chiral species grows large. Such techniques have been introduced by R. May to study the stability of ecosystems, but it is its first application to analyze the stability of the racemic state of a reaction network. To show the practical applicability of this idea, we also proposed a generalization of the Frank model with many chiral and achiral species, and we characterized its stability both analytically and numerically.
Concluding remarks
Our work suggests that the transition to homochirality in large chemical networks becomes robust and independent of many details of the reaction network. "In the end, the fact that prebiotic systems are messy from a chemical point of view, with a complex composition containing a myriad of strongly interacting organic species, is not an obstacle, but is precisely the driving force which makes the transition to homochirality more likely," concludes D. Lacoste.
Perhaps Darwin was not so wrong when he imagined that life could have started in a warm little pond. Indeed, such a setting has many attractive features: It suggests a place where molecules could accumulate and a large number of interacting chemical species would self-organize and cooperate to access to energy, and eventually build together a complex and homochiral chemical network.
This story is part of Science X Dialog, where researchers can report findings from their published research articles. Visit this page for information about ScienceX Dialog and how to participate.
More information:
Gabin Laurent et al, Emergence of homochirality in large molecular systems, Proceedings of the National Academy of Sciences (2021). DOI: 10.1073/pnas.2012741118
G. Laurent is doing his PhD under the supervision of D. Lacoste, on physical models for the origin of life, in particular the origin of homchirality. D. Lacoste is a CNRS researcher from ESPCI and PSL University in Paris, and P. Gaspard is a professor from Université Libre in Brussels.