Atom-economy and time-economy synthesis of diclofenac sodium in a desktop micro-chemical plant
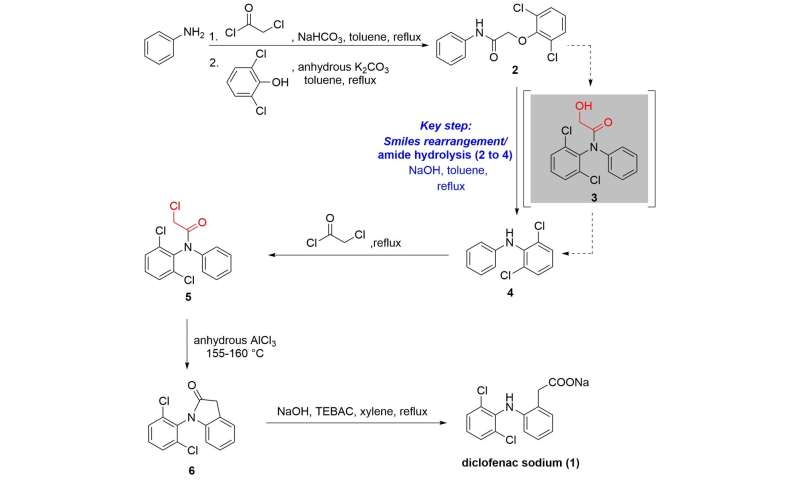
In recent years, continuous flow chemistry has evolved into a powerful platform for molecular assembly that is making an impact on organic synthetic chemistry. Unlike conventional batch method, chemical transformations are performed in small-diameter flow reactors under rigorously controlled conditions. The small characteristic scale of flow reactors and hence the high surface-to-volume ratio enable efficient mixing, excellent heat and mass transfer performances, and narrow residence time distribution. These allow reactions to be carried out at close to intrinsic reaction kinetics, thus assuring better control of reaction selectivity, higher efficiency and improved safety profile over related batch processes. Furthermore, the low inventory volume combined with the fact that the reaction is resolved along the length of the reaction channel has given rise to a novel platform that can handle hazardous/toxic materials, sensitive reagents and unstable intermediates, opening up a new scenario for assessing new chemical transformations and synthetic routes that are difficult (or even impossible) to achieve in batch mode. Therefore, there has been a growing trend recently in the fine chemical and pharmaceutical industries toward continuous flow chemistry in order to develop more efficient, safer and more sustainable chemical processes, where labor-/resource-intensive and inefficient batch synthetic approach still dominates and environmental impacts have been higher than for other industries.
Diclofenac sodium [(1) in Figure 1] is a nonsteroidal anti-inflammatory drug (NSAID) of the phenylacetic acid class widely-used in the treatment of painful and inflammatory diseases of rheumatic and certain non-rheumatic origin thanks to its fast and efficient absorption, potent effect, limited side effects, small individual differences and short elimination half-life. It inhibits the biosynthesis of the prostanoids that causes inflammation, pain and pyrexia in the body by binding with both the cyclooxygenase-1 (COX-1) and cyclooxygenase-2 (COX-2) enzymes. It is available in a number of administration forms which can be given orally, rectally or intramuscularly. The drug has been listed on 74 National Essential Medicines Lists and ranked as the eighth largest selling drug in the world. Although diverse synthetic routes have been developed for the preparation of diclofenac sodium since its first synthesis by Alfred Sallmann and Rudolf Pfister from Ciba-Geigy AG (Switzerland, now Novartis AG) in 1965, the current industrial method for the synthesis employs 2,6-dichloro-N-diphenylaniline [(4) in Figure 1] as a key intermediate, because the sensitive amide hydrolysis of hydroxyacetyldiphenylamine (3 to 4) in the Smiles rearrangement of phenoxyacetamide (2 to 3) is inevitable in the batch process (Scheme 1). Hence this leads to the adoption of one-pot Smiles rearrangement of 2 and hydrolysis of 3 to form 4 in the conventional batch approach (Scheme 1). This is robust, however, the amide hydrolysis of 3 removes a C2 unit as waste in the form of 2-hydroxyacetic acid sodium salt, and afterward, a new C2 group requires to be introduced again using highly corrosive and toxic chloroacetyl chloride. As a consequence, the unavoidable amide hydrolysis is an extra step in this traditional batch process that not only causes waste and reduces efficiency, but also leads to laborious and cumbersome operations.
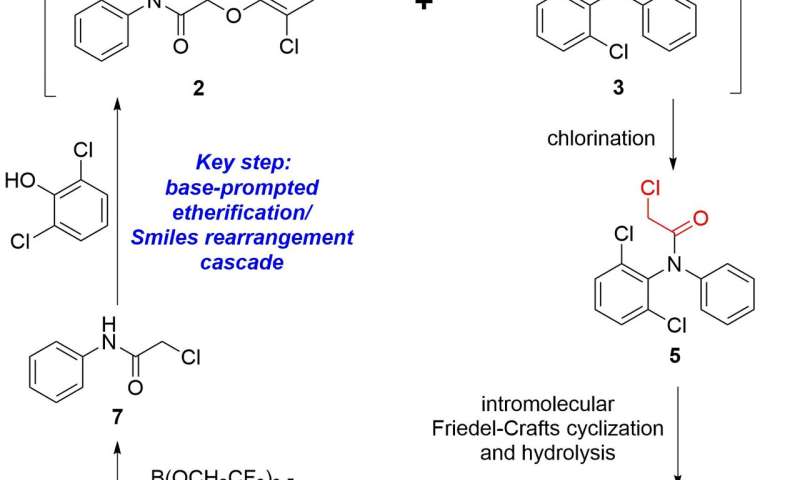
In this context, the group of Fener Chen from department of chemistry, Fudan University, China have developed a six-step continuous flow synthesis of diclofenac sodium from commercially available aniline and chloroacetic acid (Scheme 2). A novel cascade etherification/Smiles rearrangement was achieved, in which the resulting 2-chloro-N-phenylacetamide [(7) in Figure 2] and 2,6-dichlorophenol are converted to a hydroxyacetyldiphenylamine (3) directly in one flow reactor without the formation of 2, 6-dichloro-N-diphenylaniline 4, which is difficult to achieve in conventional batch method. The sensitive amide hydrolysis of hydroxyacetyldiphenylamine (3) was perfectly restrained under precisely-controlled flow conditions, thus avoiding the generation of equal equivalent of 2-hydroxyacetic acid sodium salt waste. Evidently, this further eliminates the need to introduce the C2 unit again that was required in current industrial batch approach. A chlorination, followed by an intramolecular Friedel-Crafts cyclization and hydrolysis then afforded the desired product. The streamlined synthesis gave an overall isolated yield of 63% with a total residence time of 205 min. Compared with the traditional batch synthesis, the key features of this flow approach to the synthesis of diclofenac sodium are high atom and time economy, improved sustainability, as well as simple operations. The continuous flow approach represents a greener and more sustainable process for its synthesis, involving only simple, low-cost and readily available materials and reagents (Figure 3).
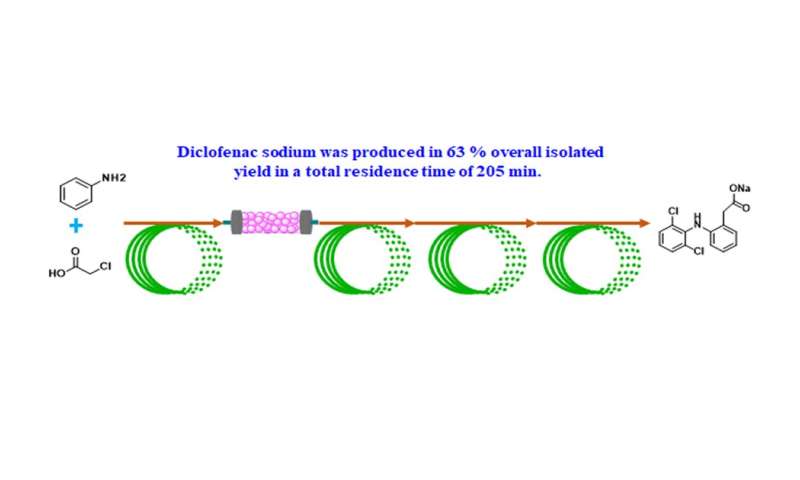
This story is part of Science X Dialog, where researchers can report findings from their published research articles. Visit this page for information about ScienceX Dialog and how to participate.
More information: Lulu Wang et al, Six‐Step Continuous Flow Synthesis of Diclofenac Sodium via Cascade Etherification/Smiles Rearrangement Strategy: Tackling the Issues of Batch Processing, Chemistry—A European Journal (2022). DOI: 10.1002/chem.202201420
Dang Cheng is a professor at the Department of Chemistry, Fudan University. He received his PhD degree in Chemical Engineering from the Institute of Process Engineering, Chinese Academy of Sciences in 2014. He then completed postdoctoral research at the Eindhoven University of Technology and Claude Bernard University Lyon 1 before joining Fudan University in 2017. His research interests include flow chemistry, continuous-flow drug synthesis, the design and development of efficient continuous-flow reactors.
Fener Chen received his M.S. in Pharmacy and his Ph.D. in Organic chemistry from Sichuan University. He joined Wuhan Institute of Technology in 1988 and was promoted to professor in 1996. In 1998, he moved to Fudan University as a full professor. His current research focuses on the development of new asymmetric catalysts, asymmetric total synthesis of natural products in continuous flow and computer assisted mechanism-based drug design (CADD). Prof. Fener Chen has been a visiting professor at numerous prestigious universities, including Washington University, King's College London, etc. He is currently a member of Chinese Academy of Engineering.