Low-temperature selective oxidation of methane by dioxygen over distant binuclear cationic centers in zeolites
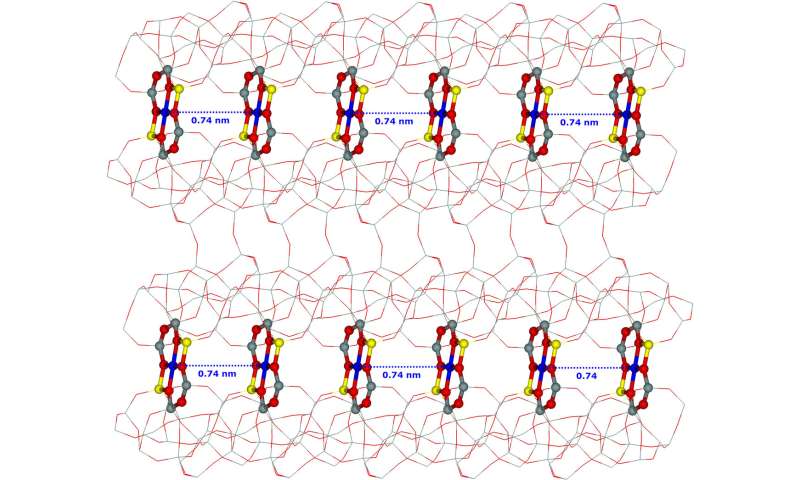
Activation of dioxygen (O2) has attracted enormous attention due to its potential for utilization of methane and applications in other selective oxidation reactions. We (Edyta Tabor, Jiri Dedecek, Kinga Mlekodaj, Zdenek Sobalik, Prokopis C. Andrikopoulos, and Stepan Sklenak of the J. Heyrovsky Institute of Physical Chemistry of the Czech Academy of Sciences, Prague) have taken a major step in enabling a cleavage of dioxygen at room temperature over distant binuclear iron species stabilized in an aluminosilicate matrix (i.e., a zeolite).
What is selective oxidation of methane, and why is it important?
Selective oxidation, in contrast to the total oxidation producing water (H2O) and carbon dioxide (CO2), allows the preparation of valuable chemical products, in our case, methanol (CH3OH) from methane (CH4). Methane, the main component of natural gas, became abundant due to the development of the shale gas technology. Nevertheless, until now, production of energy (electricity and heat) and hydrogen represent the main utilization of the methane production. Therefore, the transformation of methane to liquid products representing energy carriers and chemical production platforms is in high demand.
The selective oxidation of methane to methanol is a promising methane-to-liquid transformation. However, only the selective oxidation of methane by dioxygen is economically feasible, and represents a promising system for the use of methane with an enormous industrial impact. Conversely, the selective oxidation of methane by hydrogen peroxide (H2O2) and nitrous oxide (N2O) is rather only of academic interest, as the price of both oxidants is higher than that of methanol.
What is dioxygen?
Dioxygen, also known as molecular oxygen, comprises 21% of the atmosphere (volume ratio). It is much cheaper than other oxidants.
What are zeolites?
Zeolites are microporous, aluminosilicate materials with cavities in the form of channels or cages, commonly used as commercial adsorbents and catalysts. The ferrierite zeolite with introduced iron cations was used in the study. Ferrierite is a naturally occurring mineral, although a commercially available synthetic ferrierite was employed.
Why is iron introduced in the ferrierite zeolite?
Iron and other transition metals (e.g., cobalt, copper, nickel) serve as redox (i.e., reduction–oxidation) centers when they are introduced into the zeolite. The metals are accommodated in cationic sites and are responsible for the redox catalytic properties of these catalysts.
What are distant binuclear cationic centers in zeolites?
Iron cations (Fe2+) are introduced into the ferrierite zeolite framework. The cations do not occupy random positions, but only certain sites (i.e., cationic sites) determined by the positions of the aluminum (Al) atoms in the ferrierite zeolite framework. These aluminum positions are fixed by the conditions of the synthesis of the ferrierite zeolite. Differently synthesized ferrierite zeolites have various aluminum positions in the zeolite framework. Some ferrierite zeolites have such aluminum positions in their framework that iron cations, after their introduction in the zeolite, form iron-iron pairs in zeolite cavities with the iron-iron distance of 0.74 nm. These iron-iron pairs correspond to the distant binuclear cationic centers.
What is the mechanism of the activation of dioxygen?
The iron cations have the required redox properties, and moreover, are well positioned to interact with dioxygen and subsequently split it into a pair of Fe=O species. The oxygen atoms of the Fe=O species are called the Alpha-oxygen atoms and they are very powerful oxidants. They are even able to selectively oxidize methane to methanol at room temperature (25 °C). Until our discovery, the Alpha-oxygen atoms have been prepared only from the very expensive nitrous oxide.
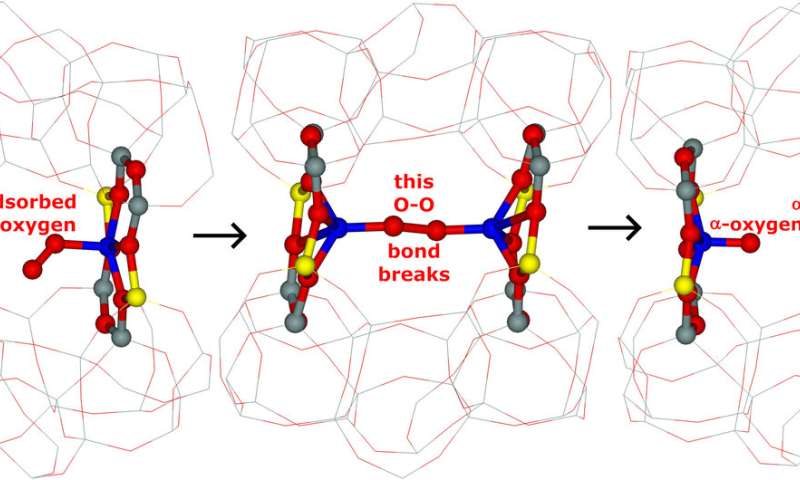
What are the advantages of the ferrierite matrix with the distant binuclear iron centers in comparison with other systems permitting the selective oxidation of methane to methanol?
Our system has an enormous practical importance as a potential industrial catalyst for methane utilization because dioxygen is used as an oxidant instead of hydrogen peroxide or nitrous oxide, but also, (i) the iron redox cycle is reversible, (ii) the active iron centers are stable under the reaction conditions, and (iii) methanol can be released to gas phase without the necessity of water or water-organic medium extraction.
In summary, our study shows that methane can be oxidized by dioxygen over distant binuclear iron species stabilized in an aluminosilicate matrix. Our results indicate a breakthrough in the development of the technology of the transformation of methane-to-liquid products representing energy carriers and chemical production platforms. Nevertheless, the application in the chemical industry requires further development of a long-term, stable system with a high activity in the methane conversion.
This story is part of Science X Dialog, where researchers can report findings from their published research articles. Visit this page for information about ScienceX Dialog and how to participate.
More information:
Edyta Tabor et al. Dioxygen dissociation over man-made system at room temperature to form the active Alpha-oxygen for methane oxidation, Science Advances (2020). DOI: 10.1126/sciadv.aaz9776
Bio:
Stepan Sklenak is a computational chemist. His research focuses on DFT calculations of zeolites to model the structure, reactivity, catalytic activity, and properties of zeolites. After finishing his Ph.D., he spent almost a decade as a postdoc and later a senior research associate at the Technion, Yale University, University of California San Francisco, and Michigan State University. After that Stepan Sklenak was awarded a Purkyne Fellowship by the Czech Academy of Sciences and became a senior scientist (2004 – present) at the J. Heyrovsky Institute of Physical Chemistry of the Czech Academy of Sciences, Prague, Czech Republic.